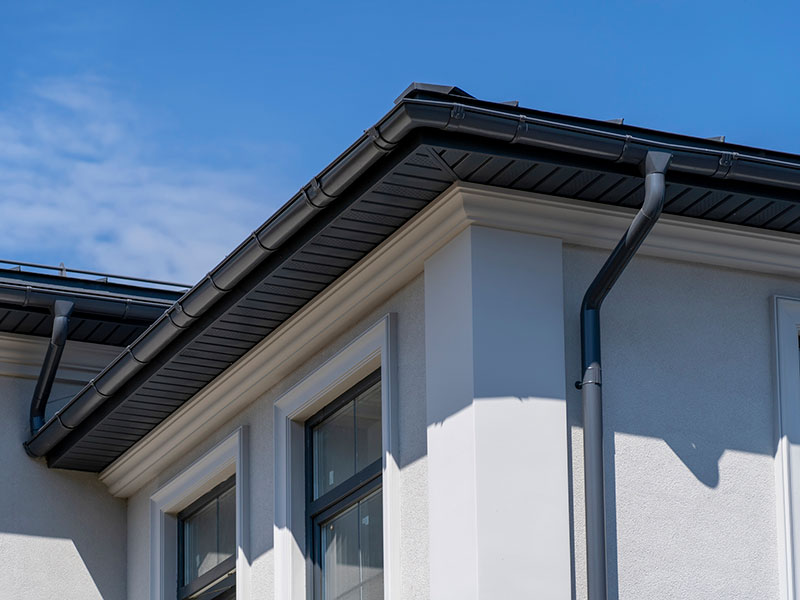
In the United States, nearly 83% of the population lives in urban areas. The combined effects of urban population growth, commercial and industrial activity, and high-water demand are well documented. The consequences of high-water demand include, but are not limited to, considerable use of surface and groundwater resources imported mostly from outside of urban boundaries; significant impact on surface water ecosystems; groundwater depletion; and substantial energy use for transporting water to urban areas. Changing climate has exacerbated water problems in urban environments, notably anthropogenic drought and flooding conditions. Three critical elements of holistic urban water management include flood control, drought management and ecosystem preservation.
To effectively cope with 21st century water management and infrastructure issues, there is critical need for a paradigm shift toward innovative approaches in urban water management. Decentralized Green Water-Infrastructure Systems (DGWIS) facilitate utilizing locally available alternative water resources (i.e. harvested rainwater, stormwater, greywater, saline water) and have the potential to integrate renewable energy sources to alleviate water scarcity and flooding, and facilitate climate change mitigation/adaptation in urban settings. DGWIS is also expected to reduce cyber-security risk compared to vulnerable large and centralized water infrastructure.
Rainwater harvesting (RWH) is a critical component of a DGWIS and resilient water management approaches in urban settings. A broad definition of RWH includes rainwater capture and use from rooftop buildings and stormwater runoff from other urban impervious surfaces such as roads and parking lots. The scope of this article is limited to the potential and merits of rooftop RWH. In urban areas, 30-40% of the impervious area is attributed to building rooftops. A 100 m2 rooftop area can collect one cubic meter of water per 1.0 cm of rain-fall. Thus, rooftop RWH can be a significant local water resource, water which is normally wasted via a stormwater drainage network. Rooftop RWH systems’ potential is determined by available water supply, which is a function of rooftop area and average monthly rainfall (minus evaporation and splash losses).
RWH for Non-Potable Use
In recent years, advances in rooftop RWH system technology have facilitated the widespread implementation of RWH systems in various public and commercial buildings for non-potable uses — toilet flushing, cooling tower make-up water, laundry, lawn and garden watering, and fountains. It is noted that non-potable uses of rooftop rainwater reduces stormwater runoff to the drainage networks and can be considered a best management practice (BMP) for stormwater runoff control.
RWH for Potable Use
Single-home or small-scale RWH for potable use is common in rural and small communities (similar to private water wells) where conventional water supply infrastructure is cost-prohibitive and/or where sufficient water resources are not available. However, potable use of rainwater in urban buildings is an emerging concept. Advances in small-scale water treatment technologies provide significant potential for rainwater treatment and potable use in urban settings.
RWH for Energy Conservation
As noted, urban (centralized) water infrastructure depends on energy for water source development, water treatment and water delivery. Captured rainwater can substitute for an equivalent volume of potable water from the public water supply system. Thus using locally available rainwater can contribute to potable (utility) water and energy saving, and therefore reduce the carbon footprint of water consumption. For example, in a case study at a single school building in Washington, D.C., based on an average annual rainfall of 100 cm and assuming a 30% water loss from the system, the rooftop of the building (about 7,711 m2) has the potential to generate as much as 5.0 x 103 m3/year of water that can be used for non-potable uses, i.e., flushing toilets/urinals and landscape irrigation. The captured rainwater substitutes for an equivalent volume of potable water from the public water supply system. For this case study site, based on estimates from electricity use data, 5.0 x 103 m3/year water delivery from a municipal utility would require 3,145 kWh of electricity per year. Instead, the RWH system uses just 776 kWh/year to meet water demand for non-potable uses. For this study site, RWH results in potential energy savings of 2,370 kWh/year. Obviously, the impact of RWH on energy and water saving would be significant if implemented on multiple buildings.
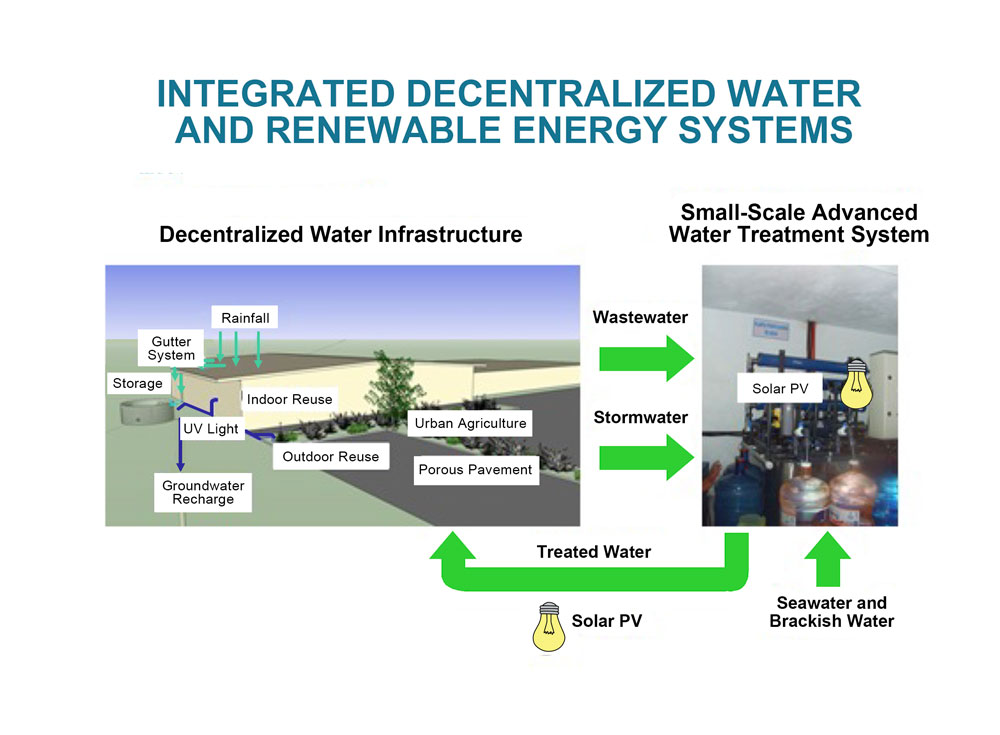
RWH for Food Security
Urban food production, i.e., urban agriculture — small backyard gardens, community gardens, greenhouses, orchards, and rooftop gardens — is considered a major component of sustainable and green urban environments, as well as providing food security and contributing to community development.
However, most urban farming systems depend on energy intensive potable utility water that could, alternatively, be substituted by captured rainwater. Geospatial analysis can be an effective tool for integrating RWH in urban agriculture. For example, in a Roanoke, Virginia, study, the National Agriculture Imagery Program (NAIP) was tapped to propose an integrated community garden and RWH system. The study identified existing urban agriculture locations, calculated adjacent rooftop areas for rainwater harvesting, potential rainwater volume and expected reduction in CO2 emissions. The illustrated example shows that RWH can be a major component of the Food, Energy, Water (FEW) Nexus, an emerging concept in urban environments.
Food Security
Urban food production, i.e., urban agriculture — small backyard gardens, community gardens, greenhouses, orchards, and rooftop gardens — is considered a major component of sustainable and green urban environments, as well as providing food security and contributing to community development. However, most urban farming systems depend on energy intensive potable utility water that could, alternatively, be substituted by captured rainwater. Geospatial analysis can be an effective tool for integrating RWH in urban agriculture. For example, in a Roanoke, Virginia, study, the National Agriculture Imagery Program (NAIP) was tapped to propose an integrated community garden and RWH system. The study identified existing urban agriculture locations, calculated adjacent rooftop areas for rainwater harvesting, potential rainwater volume and expected reduction in CO2 emissions. The illustrated example shows that RWH can be a major component of the Food, Energy, Water (FEW) Nexus, an emerging concept in urban environments.
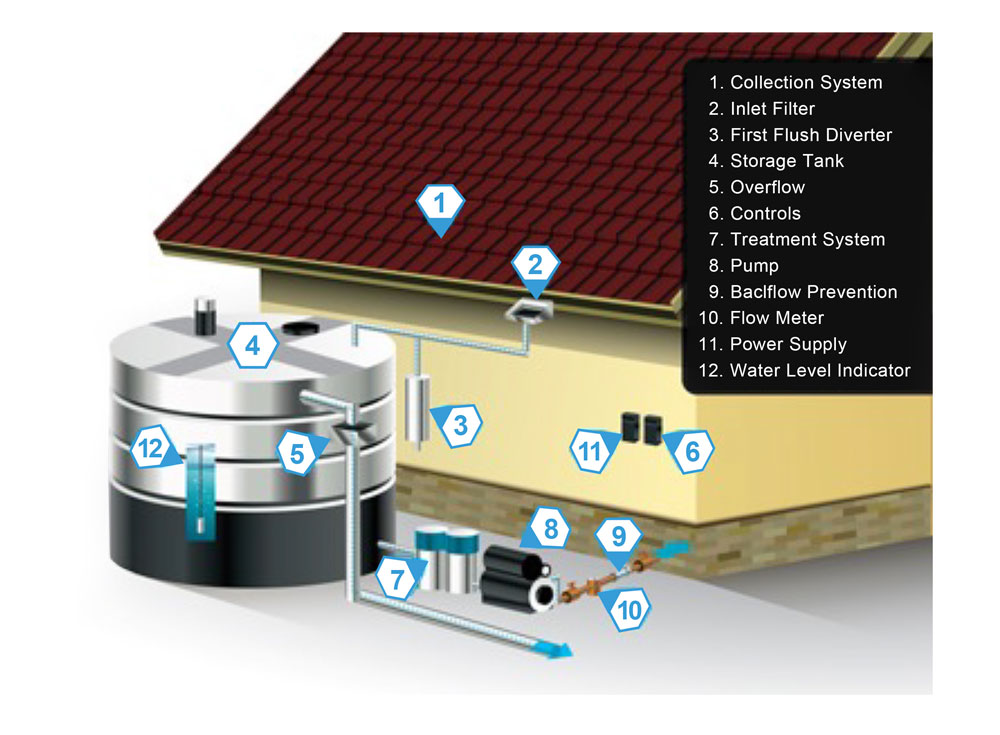
RWH – Impediments and Futuristic Solutions
There is great potential for rainwater use at multiple scales (e.g., a single building to a neighborhood community level) to achieve sustainable and resilient water management. However, RWH systems are not coordinated across water management sectors and, at present, there are barriers to widespread adoption of RWH systems. First, ambiguous guidelines among state and local governments are significant impediments in implementing local, regional and nationwide RWH systems. Second, the operation and maintenance of the RWH system in an urban setting is perceived as cost-prohibitive. Recently, life cycle impact assessment (LCIA) and benefit-cost analysis (BCA) to quantify the true benefits of RWH in terms of environmental and financial impacts have gained popularity and results are published in academic journals. A RWH system’s LCIA analysis results depend on climate factors, geography and urban/local characteristics. As an innovative strategy, forming urban RWH cooperatives for rainwater sharing, system maintenance, and financing for inspection and water testing can provide a promise for widespread implementation of RWH systems in urban settings.
The noted RWH challenges provide significant opportunities for cross-disciplinary RWH education and research development and initiating collaborative efforts among the water resources managers, academia, water industry, municipalities, legislators, environmental groups and citizens for implementing resilient water management strategies in cities of the future.
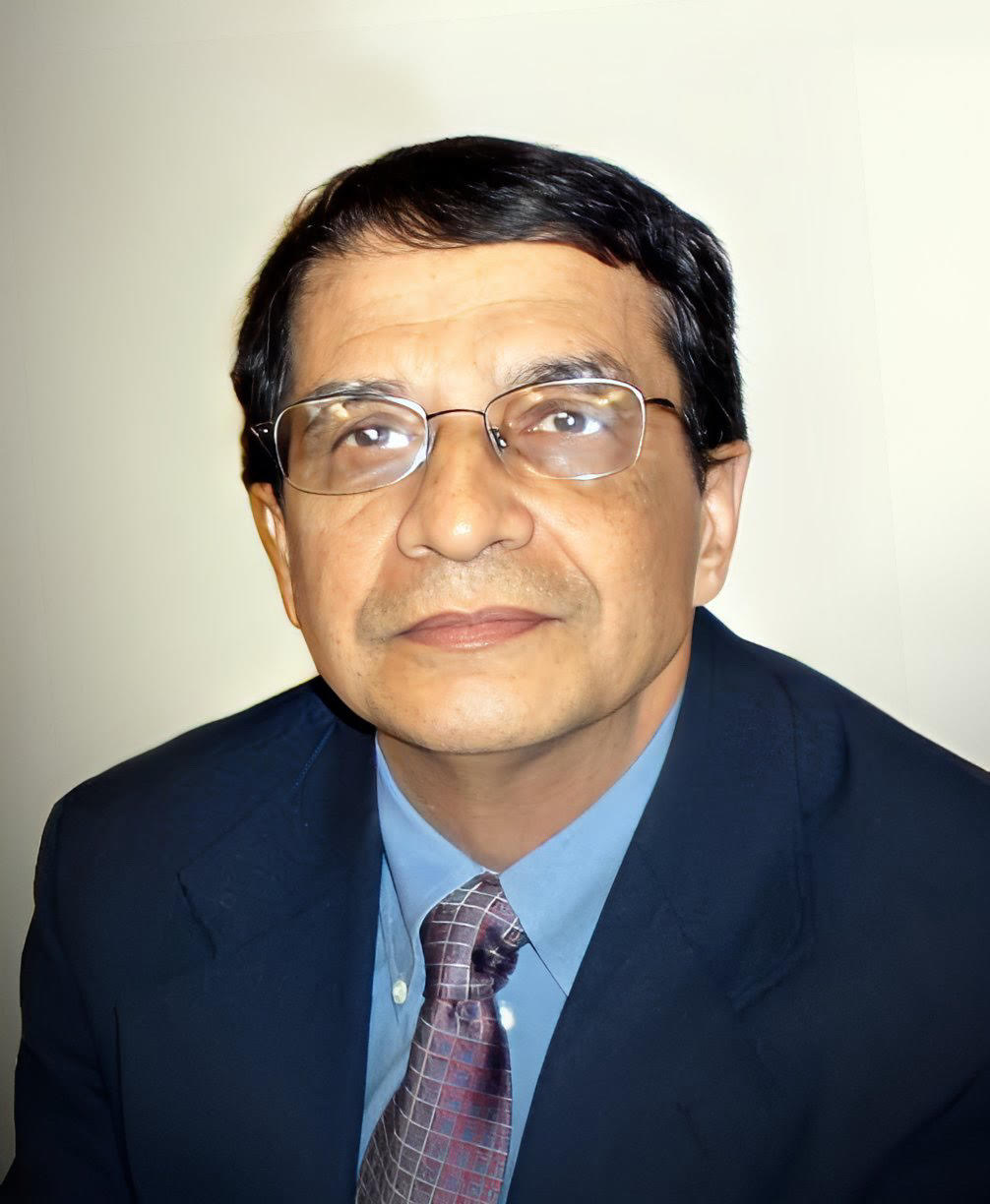
Dr. Tamim Younos
Dr. Tamim Younos, a water resources scientist, is founder and president of the Green Water-Infrastructure Academy, a nonprofit organization. He earned a doctoral degree in urban and environmental engineering from the University of Tokyo. He is a former research professor of water resources at Virginia Tech and serves as an adjunct professor of water resources at the University of the District of Columbia. He has published more than 160 research and technical articles and edited 10 books on water science and management topics. His major recognitions include: American Rainwater Catchment Systems Association (ARCSA) Lifetime Achievement Award; American Water Resources Association (AWRA) Icko Iben Award; Friend of UCOWR (Universities Council on Water Resources) Award; Fulbright Scholar (Specialist) Award; and Japan Society for Promotion of Science Fellow Award. Dr. Younos serves on the ARCSA Foundation Board of Directors (2017- present).
Last modified: March 20, 2024